The revolutionary potential of CAR-T cell therapy
Chimeric Antigen Receptor (CAR)-T cell therapy has transformed cancer immunotherapy, particularly for patients who do not respond to traditional therapies. With six CAR-T therapies approved by the Food and Drug Administration and over 1,000 active clinical trials since August 2023, CAR-T therapy offers unprecedented hope. Beyond cancer, CAR-T therapy also holds potential for treating other conditions like senescence-associated diseases, cardiac fibrosis, HIV, and autoimmune disorders.
CARs are synthetic receptors engineered to perform T-cell-activating functions by redirecting T cells to recognize and eliminate cells expressing specific target antigens. After genetically modified T cells are infused into research subjects or patients undergoing CAR-T cell immunotherapy, they begin to express CARs that target the specified antigen. The versatility of CAR-T cell therapy arises from the modular design of the receptor itself, which consists of four distinct regions: an extracellular domain for antigen recognition, a hinge region that provides flexibility and length to the CAR, a transmembrane domain that anchors the CAR to the T-cell membrane, and one or more intracellular signaling domains that activate the T cells (see Sterner and Sterner, and Sadelain, M. et al.). This modularity enables customization of CAR-T cells and addition of novel functionalities that are absent in regular T cell receptors (TCRs), enabling each component of the receptor to be fine-tuned for precise targeting.
Overcoming challenges for solid tumor treatment
Despite its potential, CAR-T cell therapy still faces significant challenges, particularly in treating solid tumors, in which CAR-T cells have not yet achieved the desired outcomes (see Sterner and Sterner, and Martinez and Moon). Solid tumors create a complex microenvironment, featuring both physical and molecular barriers, that complicate the effective use of CAR-T.
To address these challenges, Xiong and colleagues, in their review titled “The physical landscape of CAR-T synapse”, published in the August 6, 2024 Issue of Biophysical Journal entitled “Biophysics of immune cell signaling” explore the biophysical characteristics that distinguish CAR-T synapses from TCR synapses. Outlined and briefly discussed below, the authors highlight how a deeper understanding of four key biophysical features could advance the effectiveness of CAR-T therapy against solid tumors.
1. Distinct spatial distribution of proteins
Unlike the stable and organized structure of the TCR immunological synapse, CAR-T cells often display a more varied and sometimes disorganized spatial pattern. The classic “bullseye” structure of the TCR synapse is characterized by a central supramolecular activation cluster (cSMAC) surrounded by peripheral SMAC, enriched with adhesion molecules like leukocyte function-associated antigen-1 (LFA-1) and Talin. This organized structure helps stabilize the synapse and lowers its activation threshold against antigens. In contrast, CAR-T synapses often contain scattered CAR microclusters that do not always converge into a cSMAC and lack a disorganized F-actin and a peripheral adhesion ring. This irregular distribution may influence how signals are transmitted and, in turn, affect the efficacy of CAR-T cells.
Although the mechanisms underlying the unconventional formation of CAR-T synapses remain to be fully elucidated, differences in domain structure and ligand-binding affinity between CAR and TCR offer valuable clues. Unlike TCRs, CARs lack the CD3ε subunit, which binds an actin regulator and connects TCR to the cortical actin network. The authors postulate that this absence may contribute to the instability of the CAR-T synapse structure. In support of this claim, a study by Chockley et al. incorporated intracellular protein binding sites into the CAR design, specifically a PDZ-binding motif, which was shown to restore synapse stability and improve anti-tumor efficacy, probably by coupling CARs to the actin cytoskeleton.
Interestingly, Xiong and colleagues highlight that although CAR-T cells exhibit similar short-tem cytotoxicity to TCR-T cells in vitro, enhancing long-term anti-tumor responses in vivo may depend on optimizing the assembly of the CAR immunological synapse to more closely resemble that of the TCR synapse.
2. Unique structural features
Next, Xiong and colleagues discuss the unique structural features of the axial dimension of the CAR-T synapse, which may also affect how CAR-T cells engage with their targets. Unlike the TCR synapse, with its uniform 11-nm intermembrane distance, the CAR-T synapse exhibits an intermembrane distance that varies from 11to >25 nm, primarily influenced by the size of the CAR-antigen-binding pair. This variation is crucial for determining the strength of CAR activation. Here, the authors reference the previously published size-exclusion model from Su and colleagues from, which shares the same principles with the kinetic segregation model explaining TCR activation. The size-exclusion model proposes that a narrow intermembrane space can exclude the bulky phosphatase CD45 from the CAR zone, thereby favoring CAR phosphorylation by the kinase Lck, which enhances CAR-T cell activation. Thus, one could tune CAR-T cell activity by tailoring the length of the hinge domain, selecting an antigen epitope with favorable locations, or increasing the size of CD45 to exclude it from the synapse.
3. The role of cell membrane topology
Topology—the shape and surface features—of the cell membrane plays a critical role in how cells sense the surrounding environment, how they move, and how they transduce signals. Understanding CAR-T cell membrane topology can thus provide insight into the dynamics of its interactions with target cells, opening avenues for optimizing CAR-T cell design.
Like other T cells, the surface of CAR-T cells features highly dynamic finger-like membrane protrusions called microvilli. These structures, measuring 50–550 nm in diameter and extending up to several microns, play an important role in signal transduction during immune responses. Microvilli become enriched with signaling molecules before antigen recognition, enabling CAR-T cells to scan target cells for antigens and initiate synapse formation. Intriguingly, microvilli in regular T cells migrate toward the cSMAC after TCR activation but remain rather static in CAR-T cells. Interestingly, reducing CAR affinity or antigen density can mobilize these microvilli, indicating that their static nature may be linked to the strength of the CAR-antigen interaction. Interestingly, cancer cells can also use microvilli to evade immune surveillance. For instance, solid tumor cells like glioma cell lines have been shown to deploy microvilli as physical barriers, shielding themselves from T cell and CAR-T cell cytotoxicity. Thus, manipulating topological features like microvilli in both CAR-T cells and target cells may represent a strategy to enhance therapeutic effectiveness. In this context, Xiong et al. highlight several state-of-the-art techniques to visualize the CAR-T synapse with increased resolution and measure and probe their biophysical properties.
4. Mechanical force transmission
Mechanical force transmission is also crucial to CAR-T cell function. The interaction between CAR and antigen, adhesion molecules, and the pushing and pulling forces generated by the actin cytoskeleton all generate mechanical forces within the synapse. The strength and duration of these forces can significantly affect CAR-T activation. This process can be seen as a delicate tug-of-war: the goal is to generate sufficient force for effective target engagement without exerting too much. Excessive force can lead to a phenomenon known as trogocytosis, in which pieces of the cancer cell membrane are transferred to the CAR-T cell, potentially attenuating CAR-T activation and thus diminishing its therapeutic effectiveness.
Mechanical forces also play a crucial role in subsequent steps of the immune response, such as the release of lytic granules essential for killing target cells. This process involves forces exerted by LFA-1-rich protrusions, subjected to F-actin-dependent pulling that facilitates granule exocytosis in specific regions within the synapse. As Xiong et al. emphasize, “although the role of mechanical force in the lytic granule exocytosis for the CAR-T synapse remains unknown, CAR-T cells likely have distinct biophysical signatures” compared to traditional T cells, suggesting that probing these forces may offer unique insights and “establish clearer connections between the core machinery and the cytotoxic activity.”
Interestingly, killing efficiency depends on the mechanics of both the killer (T cell) and the target (cancer cell). The stiffness of the cancer cell cortex plays a key role here: softer cancer cells are more resistant to T cell–mediated killing, whereas drug treatments that increase membrane stiffness enhance T cell–mediated cytotoxicity. As suggested by the authors here, it would be noteworthy to assess whether the stiffness of the cancer cell cortex also affects CAR-T cell cytotoxicity.
Finally, Xiong et al. address the biophysical role of the glycocalyx, a dense layer of glycans, glycoproteins, proteoglycans, and glycolipids found on the cell surface. Although its precise thickness on immune cells remains largely unknown, the glycocalyx undoubtedly acts as a physical barrier that may hinder immune access to target antigens. CAR-T cells must penetrate this bulky glycocalyx, overcome its elastic resistance, and form stable ligation with target antigens, probably using the same microvilli-based mechanism as T cells. Exploring CAR-T cell design to leverage these mechanical forces or modifying the glycocalyx itself could open new avenues for enhancing CAR-T cell therapy.
Conclusions
To address current challenges limiting the broader application of CAR-T cell therapy in solid tumors, this review explores key physical features—protein distribution, membrane topology, and force transmission—that distinguish CAR-T synapses from TCR synapses. The authors highlight biophysical studies that reveal the disorganized protein pattern, variable intermembrane distance, and distinct mechanical responses of the CAR-T synapses. These features are all crucial in regulating CAR-T cell activation, suggesting that a deeper understanding of these properties may help design mechano-responsive CARs and thereby enhance therapeutic efficiency. However, as the authors emphasize, several open questions remain. Can the two-membrane, three-compartment CAR-T synapse be reconstituted in vitro to reveal mechanisms controlling protein organization and signal transduction? How do accessory receptors modulate the CAR-T synapse? And what role do environmental factors, such as shear force and stiffness, play in dictating synapse properties?
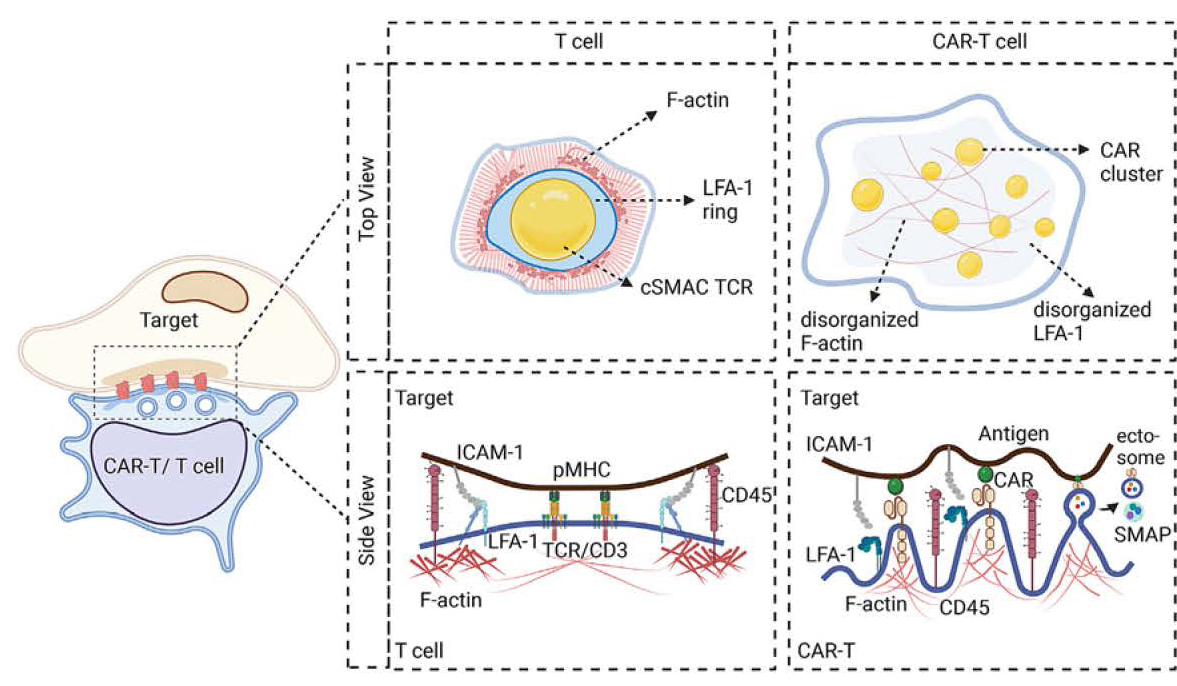
Figure 1. Schematic illustrating key characteristics of T cell versus CAR-T cell synapses. The cell (left) represents the CAR-T/T cell interacting with its target cell, highlighting the synapse (inset). The top view compares the “bullseye” cSMAC structure of the classic TCR synapse compared to the scattered CAR microclusters enriched with proximal signaling molecules found in the CAR-T synapse. The side view depicts the molecular components of each synapse, highlighting the uniform intermembrane space in the TCR synapse versus the varying width of the CAR-T synapse, influenced by CAR and antigen molecules. Adapted from Xiong et al., 2024.