September is National Sickle Cell Awareness Month in the United States. Sickle cell disease is an inherited blood disorder that affects approximately 100,000 Americans and millions worldwide. It is particularly common among people whose ancestors come from Sub-Saharan Africa, South America, Cuba, Central America, Saudi Arabia, India, and Mediterranean countries such as Turkey, Greece, and Italy.
To recognize the awareness month, we spoke with BPS member George Em Karniadakis, Brown University, and his collaborators Xuejin Li, Brown University, and Ming Dao, MIT, about their research related to sickle cell disease. Their research was also featured on the cover of the July 11, 2017, issue of Biophysical Journal.

What is the connection between your research and sickle cell disease?
Sickle cell disease (SCD) is the first identified molecular disease affecting more than 270,000 new patients each year. Our interests are in modeling multiscale biological systems using new mathematical and computational tools that we develop in our teams at Brown University and MIT in conjunction with carefully selected microfluidic experiments at MIT. We have an ongoing NIH-funded joint project that focuses on developing such validated predictive models for the sickle cell disease (SCD). In this project, with close collaboration between clinicians, experimentalists, applied mathematicians and physical chemists, we have been developing new predictive patient-specific models of SCD, linking sub-cellular, cellular, and vessel-level phenomena spanning across four orders of magnitude in spatio-temporal scales. So far we have developed a validated patient-specific and data-driven multiscale modeling approach to probe the biophysical mechanisms involved in SCD from hemoglobin polymerization to vaso-occlusion.
Why is your research important to those concerned about sickle cell disease?
SCD is one of the most common genetic blood disorders that can cause several types of chronic and acute complications such as vaso-occlusive crises (VOC), hemolytic anemia, and sequestration crisis. It is also the first identified molecular disease (as early as 1947 by Linus Pauling), and the underlying molecular cause of the disease has been understood for more than half a century. However, progress in developing treatments to prevent painful VOC and associated symptoms has been slow. Consequently, we have been developing a “first-principles” multiscale approach that can handle the disparity of molecular, mesoscopic and macroscopic phenomena involved in SCD simultaneously. Such simulations could potentially answer questions concerning the links among sickle hemoglobin (HbS) polymerization, cell sickling, blood flow alteration, and eventually VOC. We hope, in turn, that these models will help in assessing effective drug strategies to combat the clinical symptoms of this genetic blood disorder.
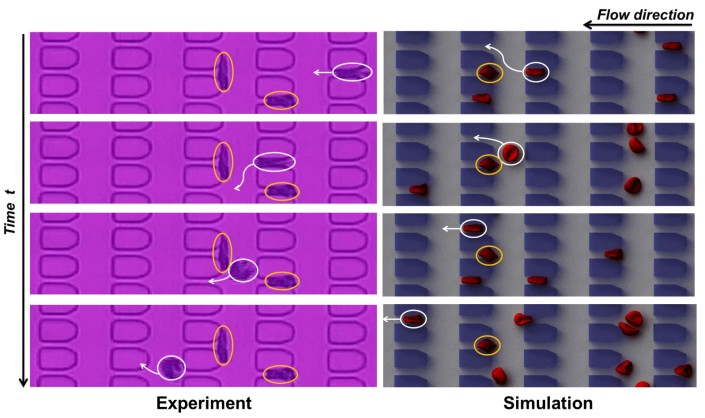
Figure 1. Dynamic behavior of individual sickle RBCs flowing in microfluidic channel. Inside the yellow circles are trapped sickle RBCs at the microgates, and inside the white circles are deformable RBCs, which are capable of circumnavigating trapped cells ahead of them by choosing a serpentine path (indicated by the white arrows).
How did you get into this area of research?
We have been working on multiscale modeling of blood disorders for more than 10 years. In the very beginning, we were interested in developing new computational paradigms in multiscale simulations, which would enable us to perform multiscale realistic simulation of blood flow in the brain of a patient with an aneurysm. We then realized that the mesoscopic modeling of red blood cells (RBCs) and hemorheology in general seems to be the most effective approach for modeling malaria and other hematologic disorders. Then, we shifted our attention to the particle-based modeling of blood flow by employing the dissipative particle dynamics (DPD) method, which can seamlessly represent the RBC membrane, cytoskeleton, cytosol, surrounding plasma, and even the parasite in the malaria-infected RBCs. We developed multiscale RBC models and employed them to predict mechanical and rheological properties of RBCs and quantify molecular-level mechanical forces involved in bilayer–cytoskeletal dissociation in blood disorder. In 2012, we started to work on SCD, after realizing that no multiscale simulation studies of SCD had been conducted before – our work is the first of its kind!
How long have you been working on it?
As we mentioned above, we have been working in this field for more than 10 years.
Do you receive public funding for this work? If so, from what agency?
Yes, we receive support from NHLBI, the institute within NIH focusing on blood disorders based on the interagency funding initiative pioneered by Dr. Grace Peng. For those who are interested in this multiscale consortium they can visit: https://www.imagwiki.nibib.nih.gov/
Have you had any surprise findings thus far?
Plenty! For example, at the vessel scale, using computer models, we have discovered that it is the soft and sticky type of RBCs that initiate the blockage process and lead to sickle cell crises and not the rigid sickle cells! This is the first study to identify a specific biophysical mechanism through which vaso-occlusion takes place. At the cellular scale, we have developed a tiny microfluidic device that can analyze the behavior of blood from SCD patients. Informed from the microfluidic experiments conducted by Dr. Ming Dao’s group at MIT, we have developed a unique patient-specific predictive model of sickle RBCs to characterize the complex behavior of sickle RBCs in narrow capillary-like microenvironment. At the sub-cellular (molecular) scale, we have developed a particle HbS model for studying the growth dynamics of polymer fibers (recent cover of Biophysical Journal). The simulations provide new details of how SCD manifests inside RBCs, which could help other medical researchers in developing new treatments.
What is particularly interesting about the work from the perspective of other researchers?
It is known that the primary cause of the clinical phenotype of SCD is the intracellular polymerization of sickle hemoglobin (HbS) resulting in sickling of RBCs in deoxygenated conditions. However, the clinical expression of SCD is heterogeneous, making it hard to predict the risk of VOC, and resulting in a serious challenge for disease management. Our data-driven stochastic multiscale models, based on particle methods, can be used to explore and understand the dynamics of collective processes associated with vaso-occlusion that links together sub-cellular, cellular, and vessel phenomena. A similar computational framework can be applied to study blood flow in other hematologic disorders, including malaria, hereditary spherocytosis and elliptocytosis, as well as other blood pathological conditions in patients with diabetes mellitus or AIDS. For example, in ongoing work we have quantified the biophysical characteristics of RBCs in type-2 diabetes mellitus (T2DM), from which the simulation results and their comparison with currently available experimental data are helpful in identifying a specific parametric model that best describes the main hallmarks of T2DM RBCs. Perhaps, the most important extension is to connect such multiscale models to all the “omics” technologies (genomics, proteomics, metabolomics, etc.) to implement the vision of precision medicine advocated both in the U.S. and around the world.
What is particularly interesting about the work from the perspective of the public?
Our studies provide new insight into what causes painful episodes in people with SCD. Using the computational models we could probe different mechanisms and validate diverse hypotheses regarding vaso-occlusion. For example, we have shown that the rigid crescent-shaped RBCs —the hallmark of SCD — do not cause these blockages on their own. Instead, softer, deformable RBCs are known as cells that start the process by sticking to arteriolar and capillary walls. The rigid crescent-shaped cells then stack up behind these softer cells, creating a sort of a traffic jam.
Currently, hydroxyurea (HU) is the only approved medication in widespread use for the treatment of SCA, and it is thought to work by promoting the production of fetal hemoglobin, which can reduce sickling rate. Using the computational models, we can now run simulations that include fetal hemoglobin, which could help in establishing better dosage guidelines or in identifying a subgroup of patients who would benefit from this treatment or proposing a different type of treatment for others.
In addition, based on our own experience and knowledge, we also presented a short review in SIAM NEWs, which provides the broader public with a general idea of computational modeling of blood disorders, including SCD. Here is the link to the review: https://sinews.siam.org/Details-Page/in-silico-medicine-multiscale-modeling-of-hematological-disorders.
Do you have a cool image you want to share with the blog post related to this research?
Yes, we have a cool image to share (figure 1). This image shows the different dynamic behavior between individual normal RBCs and sickle ones in microfluidic flow. Normal RBCs are round and flexible, and easily change shape to move through even the smallest blood vessels. Under deoxygenation, RBCs undergo sickling can be hard, sticky, and abnormally shaped, so they tend to get stuck at the microgates and block the blood flow. Once the adjacent microgates in the flow direction (from right to left) are blocked, the deformable RBCs (one is highlighted in white circle) appear to take a preferred path, i.e., they twist and turn along a serpentine path (as indicated by the white arrows) once they spot trapped sickle cells (one is highlighted in yellow circle) ahead of them.